Mission
The UTRGV Department of Physics and Astronomy mission is to pursue excellence in research and education across Physics and Astronomy, including multi-disciplinary areas, in a diverse, inclusive, and nurturing environment with emphasis on transformative impact on traditionally underrepresented minorities, and to serve the community, the profession, and the society at large through dissemination of scientific knowledge and promotion of scientific literacy.
Vision
The UTRGV Department of Physics and Astronomy envisions empowering our students to become successful leaders, innovators, and educators through world-class education and research, while nurturing them to be conscientious citizens with sensitivity towards diversity and inclusiveness, performing impactful research and engaging the local community in science.
Academic Programs
Bachelor's
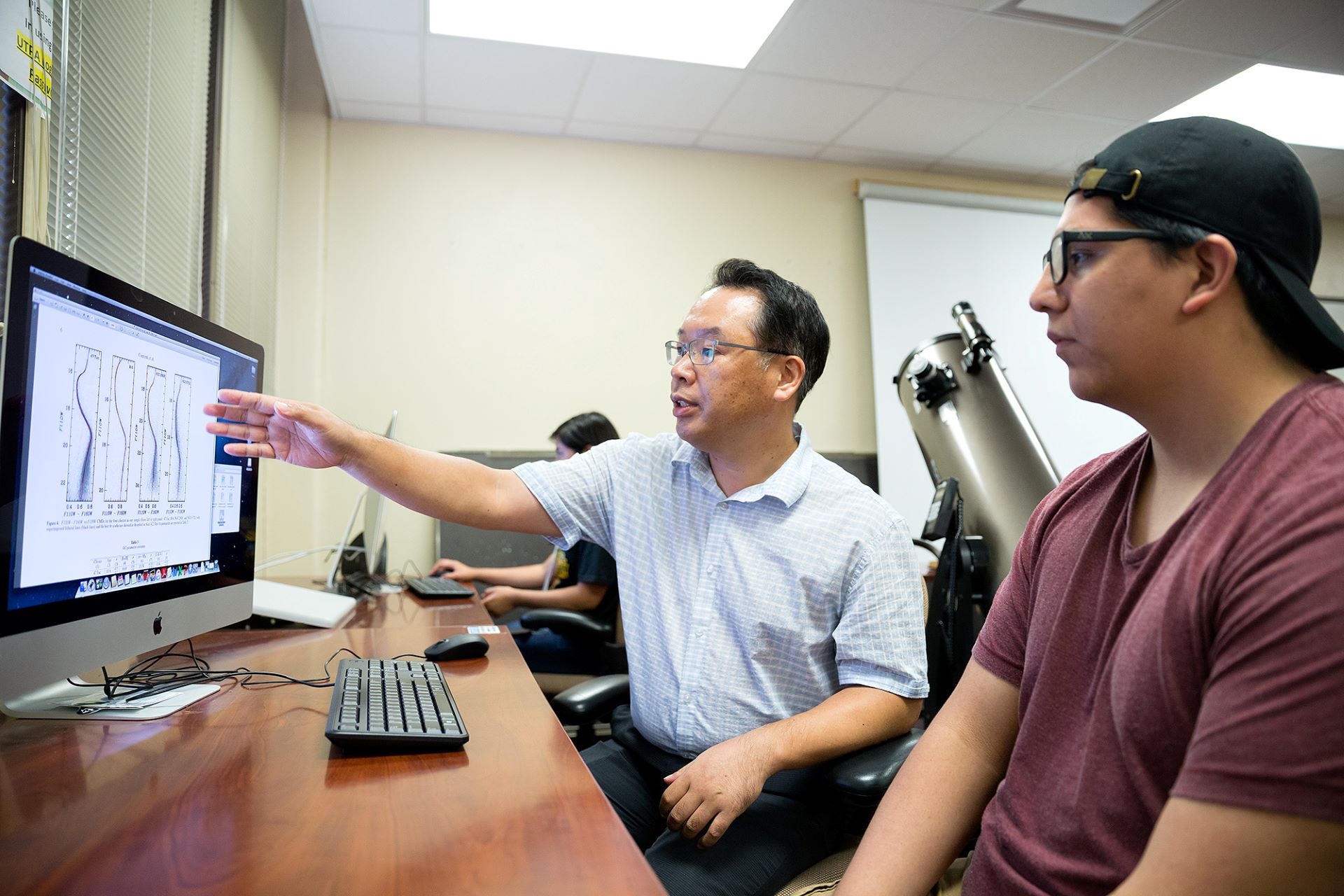
Bachelor's Degree
Physics (BS)
Bachelor of Science in Physics
Program Modality: Campus
The Bachelor’s degree in Physics provides students with a solid understanding of the fundamental laws that can be applied to a wide array of scientific and engineering fields, including gravitational wave technology, computational physics, and biophysics. The program offers two concentrations that prepare students for the pursuit of graduate studies.
Concentrations:
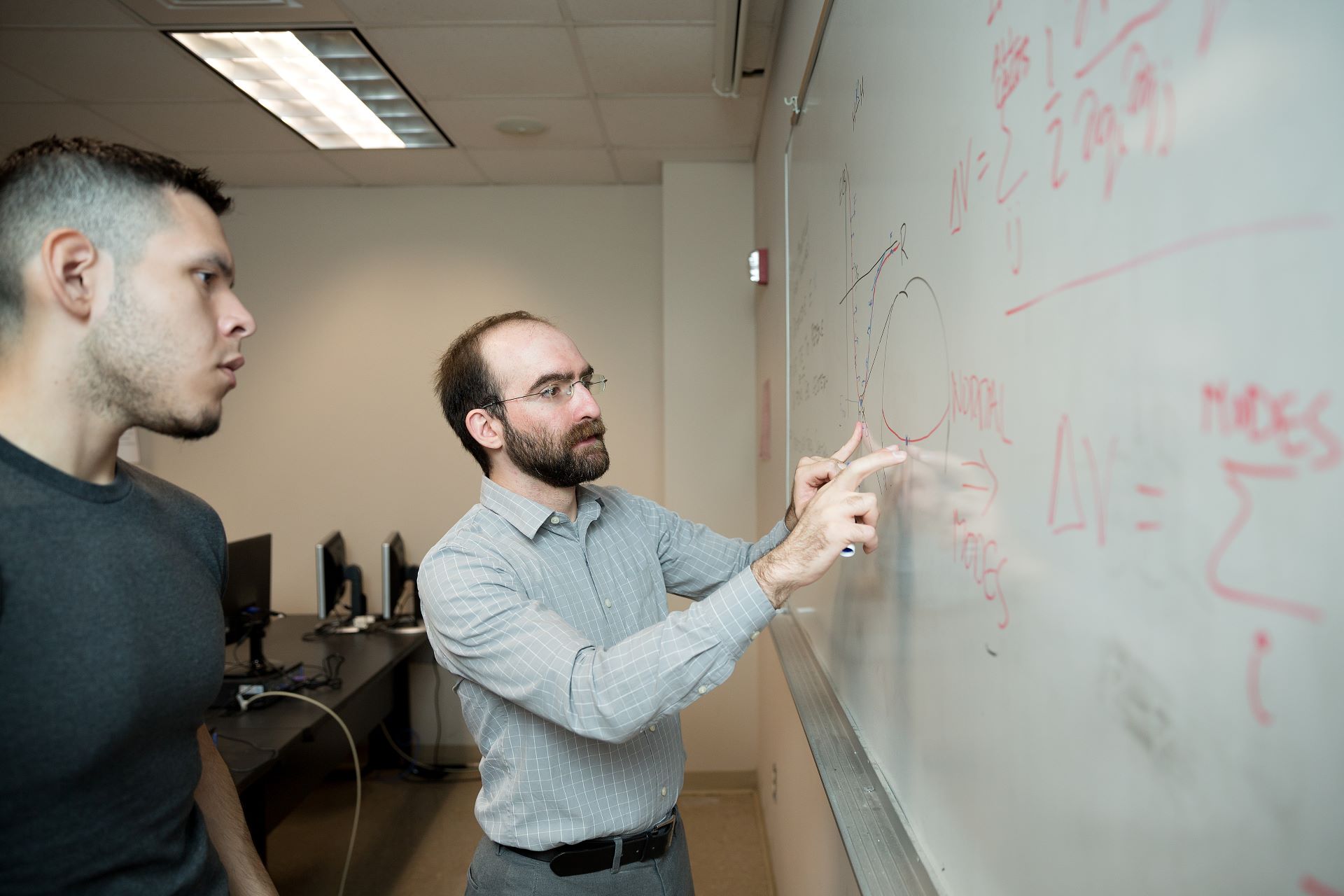
Bachelor's Degree
Physics - Teacher (BS)
Bachelor of Science in Physics (Teacher Certification)
Program Modality: Campus
The Bachelor’s degree in Physics with Teacher Certification prepares students to become proficient in the physics field while learning how to educate the future generation of scientists. The program provides a pathway to teacher certification.
UTRGV is approved by the State Board of Educator Certification to recommend students who complete all requirements of this degree for teaching certification.
Master's
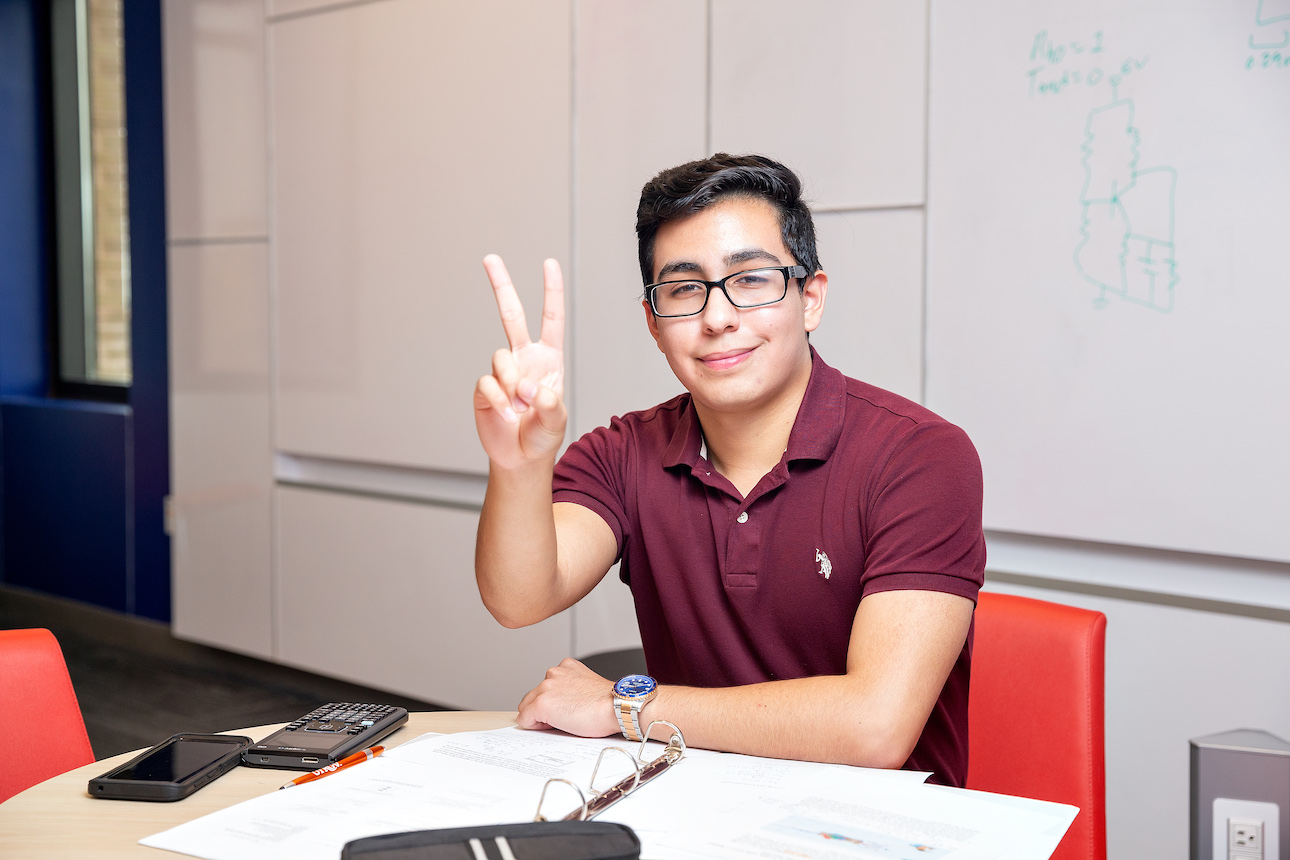
Master's Degree
Physics (MS)
Master of Science in Physics
Program Modality: Campus
The Master’s degree in Physics is designed for students who want to pursue excellence in research and education in physics to serve the community, the profession, and society at large through the dissemination of scientific knowledge and promotion of scientific literacy. Program graduates are prepared to conduct research in areas of matter and energy interaction, astronomy, astrophysics, and other physics areas.
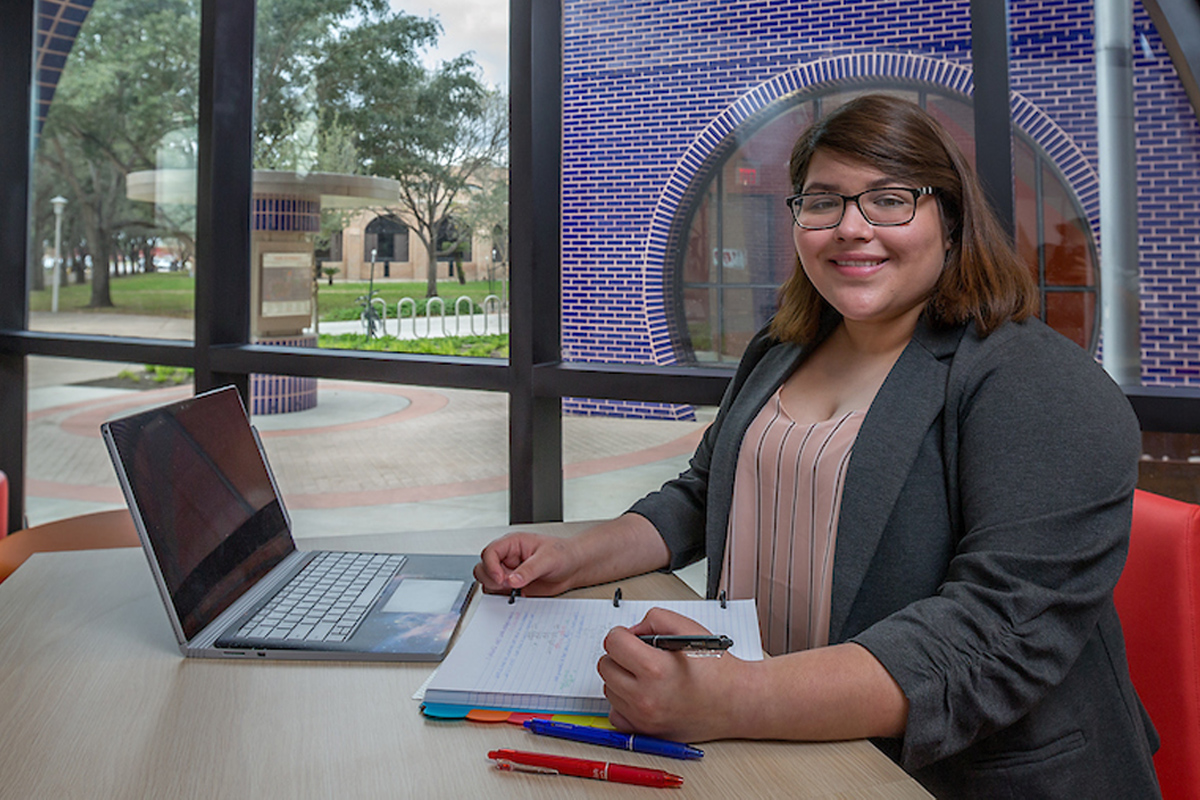
Master's Degree
Physics (MSIS)
Master of Science in Interdisciplinary Studies with a Concentration in Physics
Program Modality: Campus
The Master of Science in Interdisciplinary Studies (MSIS) program with a concentration in Physics is a 30-hour program tailored to STEM teachers and STEM majors seeking comprehensive interdisciplinary training and research experiences. Depending on your career goals, you will select 18 hours of physics coursework, complemented by 6-12 hours in fields such as Education, Chemistry, Computer Science, and Mathematics. The MSIS program offers both thesis and non-thesis tracks to cater to your academic preferences.
This unique program equips you with the scientific knowledge and interdisciplinary research skills necessary to thrive in the ever-evolving professional landscape. Graduates emerge with a robust foundation in physics, mathematics, chemistry, instructional technology, computer science, and STEM education and research, positioning them as highly competitive professionals in today's dynamic workforce.
Doctorate
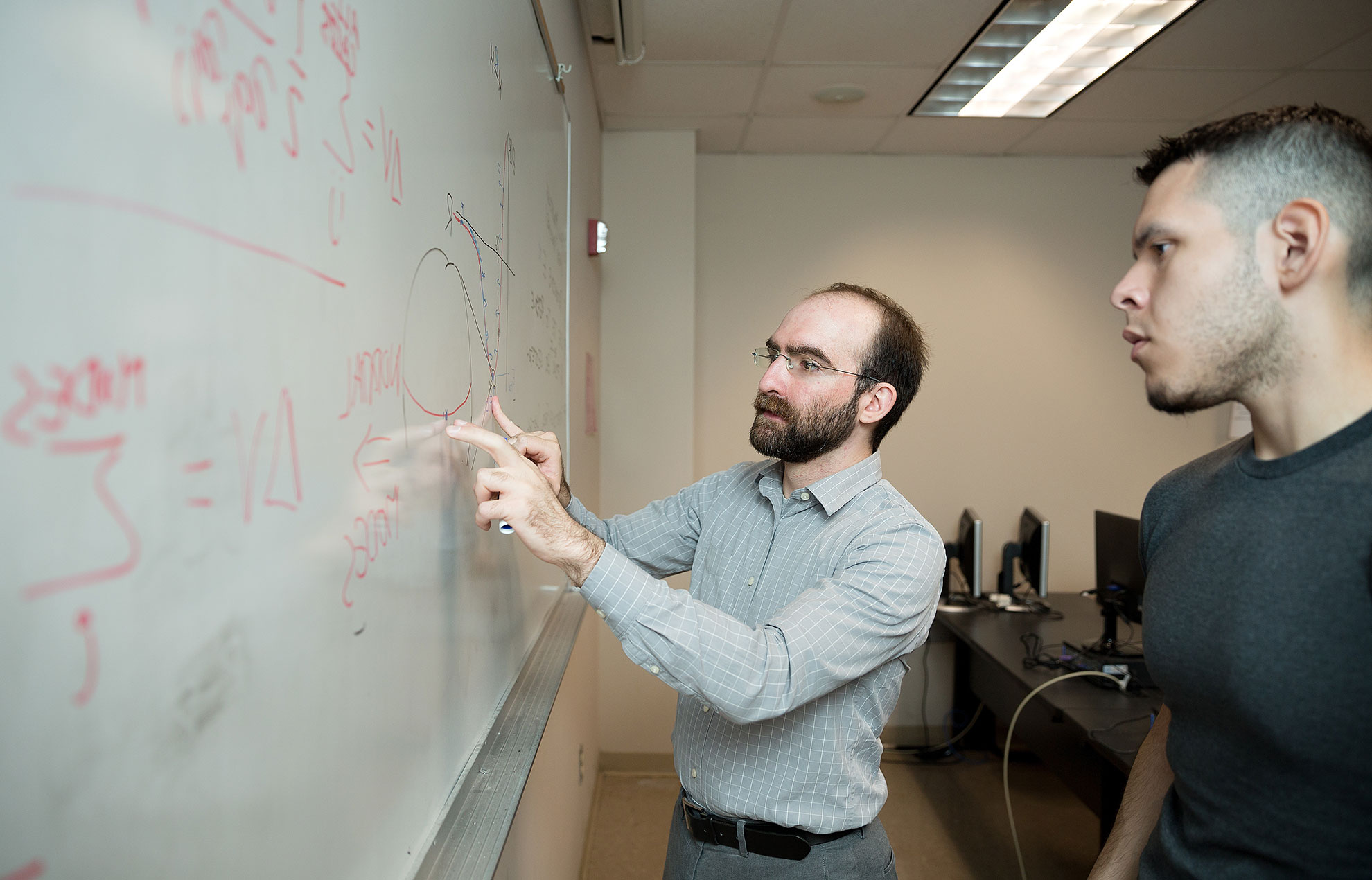
Doctoral Degree
Physics (PhD)
Doctor of Philosophy in Physics
Program Modality: Campus
The Doctoral degree in Physics provides students with a broad background in classical and modern physics. Modern physics has entered a new era of expanding quantitative understanding of large-scale and multidisciplinary complex systems, impacting science, technology, medicine, and the economy.
The program trains professionals who can develop research-based solutions in the areas of gravitational wave astronomy, optical and radio astronomy, optical physics, nanophysics, and materials physics, condensed matter physics, high-energy physics, biophysics, biomedical physics, and physics education.